O1. Nanostructured cellulose interface preparation, the base of sensor (2020-2021)
This objective is considered the first step of the overall biointerface process. Several types of cellulosic samples, including cotton linters, microcrystalline, never-dried sulfite pulp, and viscose, were tested as potential sources to prepare cellulose-based derivatives, able to be used in homogeneous systems.
The chosen derivatization procedure implies the chemical oxidation using 2,2,6,6-tetramethylpiperidine-1-oxyl (TEMPO) radical, sodium hypochlorite, and sodium bromide. Following this treatment, each cellulose sample was processed in such a way as to maximize the content of water-soluble fractions. Therefore, for each cellulose type (except viscose), we could isolate three oxidized samples, see Fig. 1.
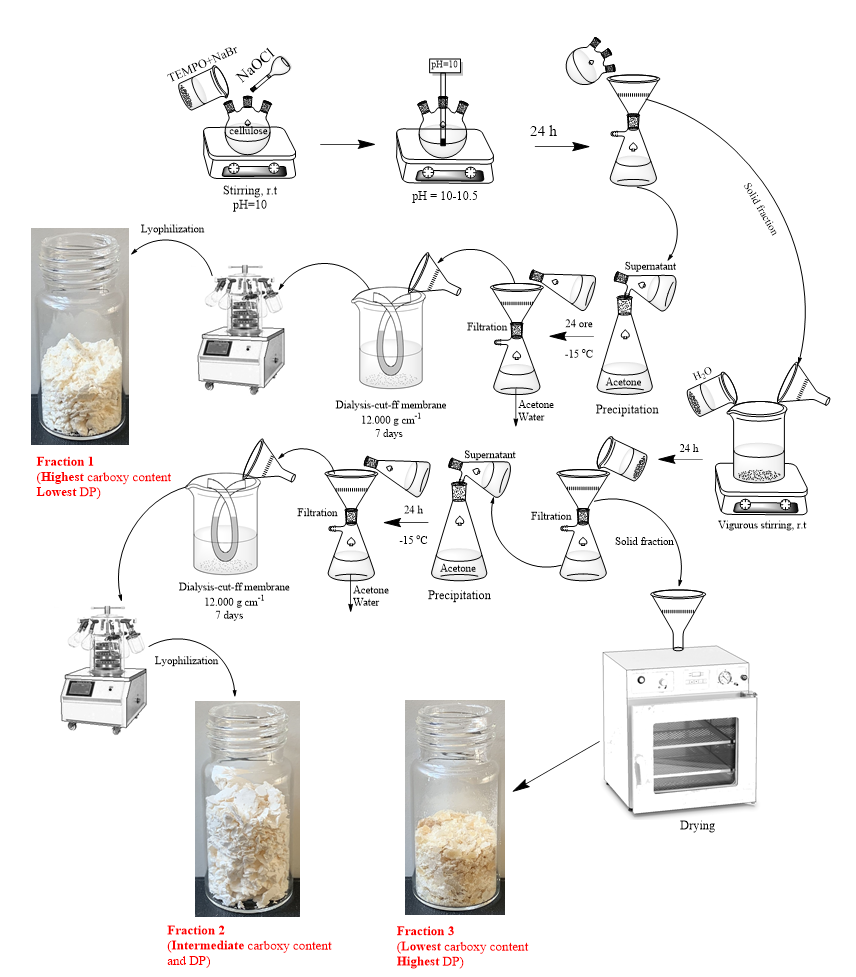
Fig. 1. General scheme for the production cycle of oxidized cellulose fractions with different water solubility.
Furthermore, Fig. 2 shows the appearance of 1% suspensions of CL, CL 1, CL2 and CL 3 samples, in several solvents, like: water, DMF, acetone, ethanol, and DMSO.
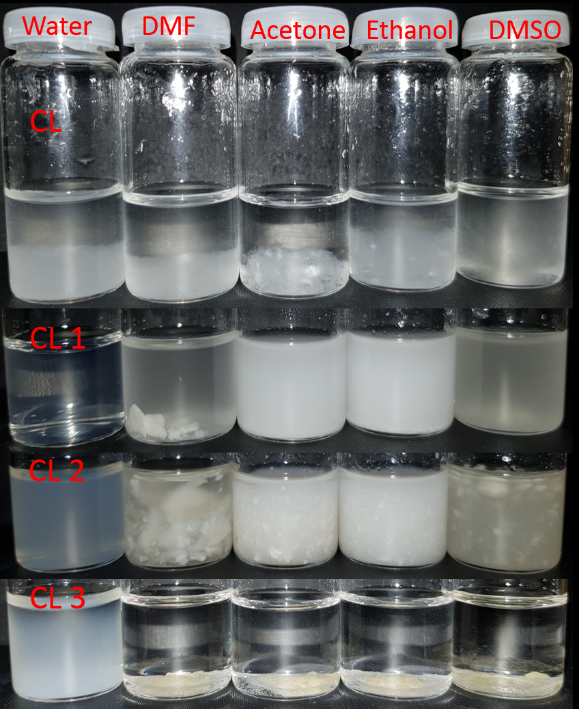
Fig. 2. Photographs of 1% suspension stability of CL and the isolated oxidized fractions (CL 1, CL 2, CL 3) in water, DMF, acetone, ethanol and DMSO.
It can be observed, that oxidized fractions reach in carboxyl groups, CL 1 and CL 2 displays a good solubility in water; The other oxidized fraction, CL 3, with less carboxyl groups incorporated, do not dissolve into water, forming only a suspension. CL 1 sample exhibit a good dispersibility also in acetone, ethanol and DMSO, but not in DMF.
The solubility limit in water of the two oxidized fractions was additionally checked by using low-voltage electron microscopy (LVEM). LVEM is a powerful transmission electron microscope able to acquire high-resolution images of biological and organic samples, combining the capabilities of the TEM, SEM and STEM techniques. As the Fig. 3 reveals, the solubility of the CL 1 sample in water is excellent until 0.8% concentration, while the sample CL 2 displays a good water solubility in the range of concentrations until 0.2%. At higher concentrations, above the mentioned values, both samples tend to form agglomerations, or clusters visible under a microscope, indicating that the solubility limit has been reached.
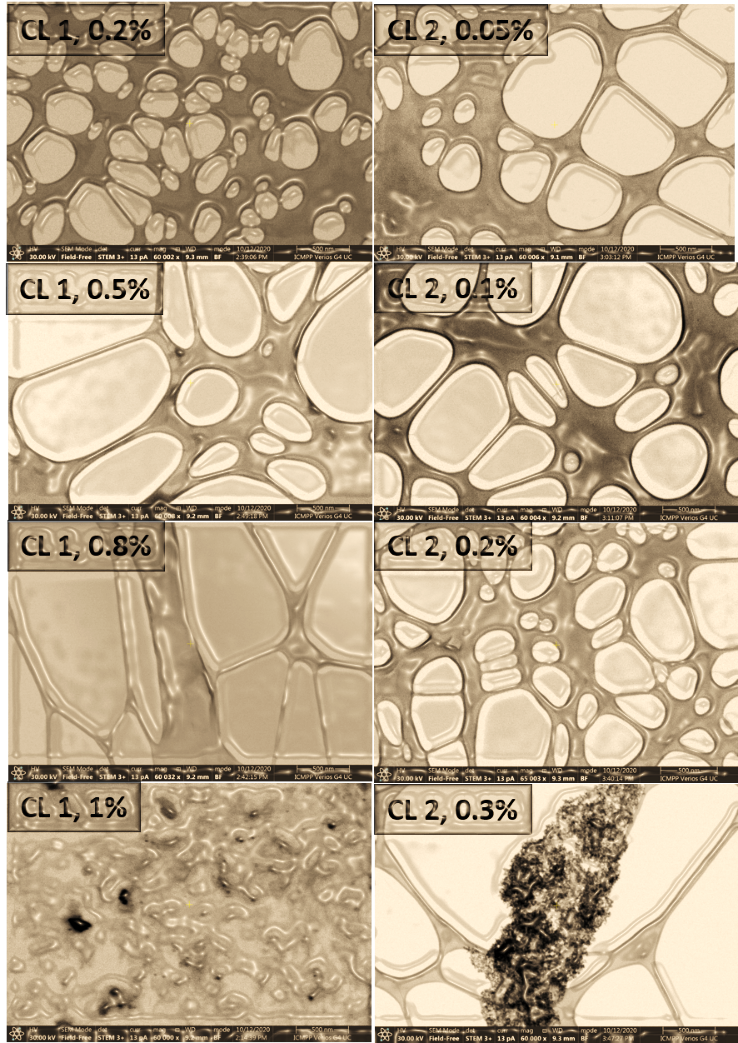
Fig. 3. STEM images of aqueous solutions of different concentrations of CL 1 and CL 2 samples.
O2. Linking the reactive sites on the sensor base surface (2021)
This step imply the use of the previously synthetized surfaces as platforms for the anchoring of binding sites for further immobilization of hIgG.
To achieve the base surface, the COOH groups previously introduced in the anhydroglucose unit of cellulose were first activated. The activation is performed by using a classical EDC/NHS coupling protocol, as depicted in the Fig. 4.
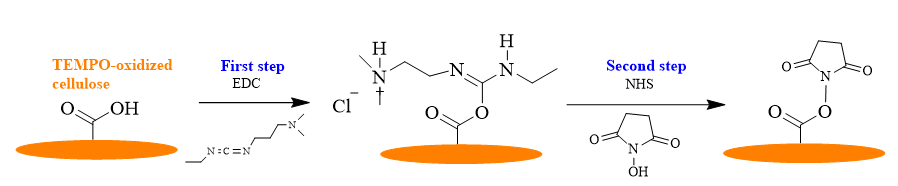
Fig. 4. Schematic representation of COOH groups activation from TEMPO-oxidized cellulose by using the EDC/NHS protocol.
The second step consist on the hexamer short peptide HWRGWV attachement onto the activated cellulose surface. The process has been monitored inside the QCM-D instrument, the resulted films being analysed by means of atomic force microscopy (AFM), Fig. 5.
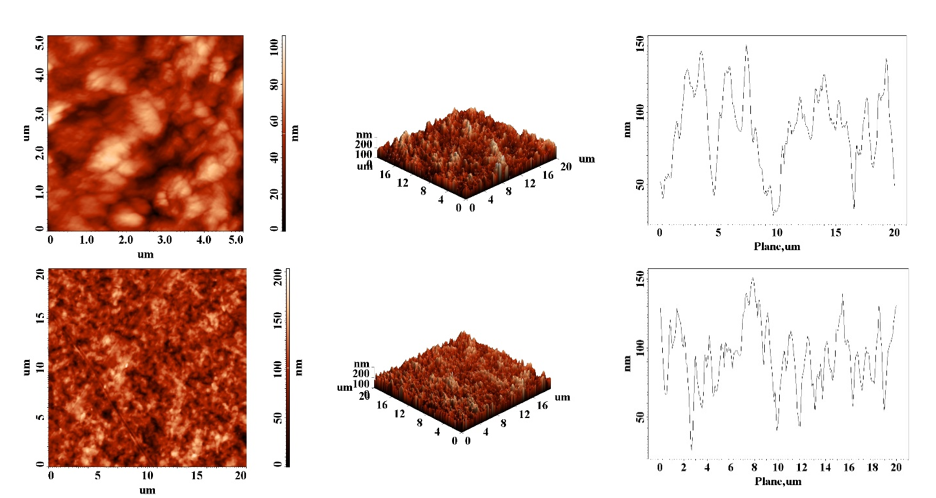
Fig. 5. Cellulose films morphology before (up) and after (down) TEMPO-oxidation, EDC/NHS activation and coupling with peptide hexamer HWRGWV.
Publications
1. R.I. Baron, S. Coseri, Preparation of water-soluble cellulose derivatives using TEMPO radical-mediated oxidation at extended reaction time, Reactive and Functional Polymers, 157, 104768, 2020. IF = 3.975
2. M.E. Culica, A.L. Chibac-Scutaru, T. Mohan, S. Coseri, Cellulose-based biogenic supports, remarkably friendly biomaterials for proteins and biomolecules, Biosensors and Bioelectronics, 182, 113170, 2021. IF = 10.618
3. I.A. Duceac, L. Vereștiuc, A. Coroaba, D. Arotăriței, S. Coseri, All-polysaccharide hydrogels for drug delivery applications: Tunable chitosan beads surfaces via physical or chemical interactions, using oxidized pullulan, International Journal of Biological Macromolecules, Volume 181, 1047-1062, 2021. IF = 6.953
4. O. Burduniuc, A.C. Bostanaru, M. Mares, G. Biliuta, S. Coseri, Synthesis, characterization, and antifungal activity of silver nanoparticles embedded in pullulan matrices, Materials, 14, 7041, 2021. IF = 3.623
Participations at scientific events
1. R.I. Baron, G. Biliuta, S. Coseri, Preparation of water-soluble carboxylated cellulose derivatives as efficient hydrogel promoters, Bucharest Polymer Conference 2nd Edition, University POLITEHNICA of Bucharest, Romania, June 10-11, 2021 - oral communication
2. I.A. Duceac, S. Coseri, Oxidized pullulan for fine-tuning a chitosan-based drug delivery system, Bucharest Polymer Conference 2nd Edition, University POLITEHNICA of Bucharest, Romania, June 10-11, 2021 - oral communication
3. A.L. Chibac-Scutaru, V. Melinte, S. Coseri, Cellulose-derived platforms for targeted technological applications, 13th International Conference on Physics of Advanced Materials, Sant Feliu de Guixols, Spain, September 24-30, 2021 - oral communication
4. R.I. Baron, G. Biliuta, S. Coseri, Hydrogels based on PVA and pullulan with self-healing behavior, Advanced Materials in Modern Medicine – International Congress ”By promoting excellence we prepare the future” Iasi, Romania, March 1–3, 2021 - poster |